Research
With our research we focus on the relationship between form, function and ecology of living and extinct animals. Our projects focus on the different ways that biomechanics of movement can limit the pathways available for evolution.
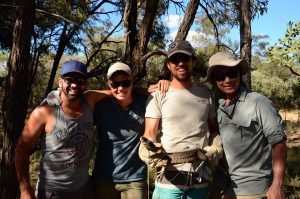
Research Interests:
We have studied morphology, metabolic rates and biomechanics and compared these to ecological characteristics and locomotory ability in a large group of lizards, the varanids. Similarly, we have studied these traits in other lizard groups, including an extensive project examining the evolution of bipedalism in dragon lizards, showing lizards were essentially popping a wheelie. Other species like the Marine Iguana, only to be found on the Galapagos Islands, are subject to our studies as well. Not only lizard shaped animals draw our attention. We also study the question why invasive species in Australia are so successful compared to native species and we look at the turning ability of dogs to figure out the biomechanics laying behind that. Turns out (da dumm) that turning in a small radius is quite difficult, as experiments on ourselves confirmed. Lastly, we don’t want to miss out on studying the iconic Australian species: Kangaroos and Koalas.

Research Methods:
As different as these animals are, so are the methods we apply to do our research. Whether it is the use of drones to film animals from above, or the use of high-speed cameras to get a high-resolution video of the locomotor performance of the animal in 2D and 3D. Whether it is the use of physical robotic models or computational muscle-skeletal modelling. Whether it is tracing animals’ movements with accelerometers and using machine learning to predict their behaviour or tracking the movements using markerless pose-estimation based on deep learning to study detailed kinematics. If kinematics alone is not enough – don’t worry! We do also record force data of animals running, climbing and hopping to investigate their movement dynamics.
All these methods help us to answer questions about the pathway and limitations of evolution, why animals move in the way they do and why they occupy the habitat they live in.
Our main research fields:
Bio-inspired Robotics
Build simplified physical, climbing and jumping robotic models to create a complete map of the adaptive landscape for a performance task
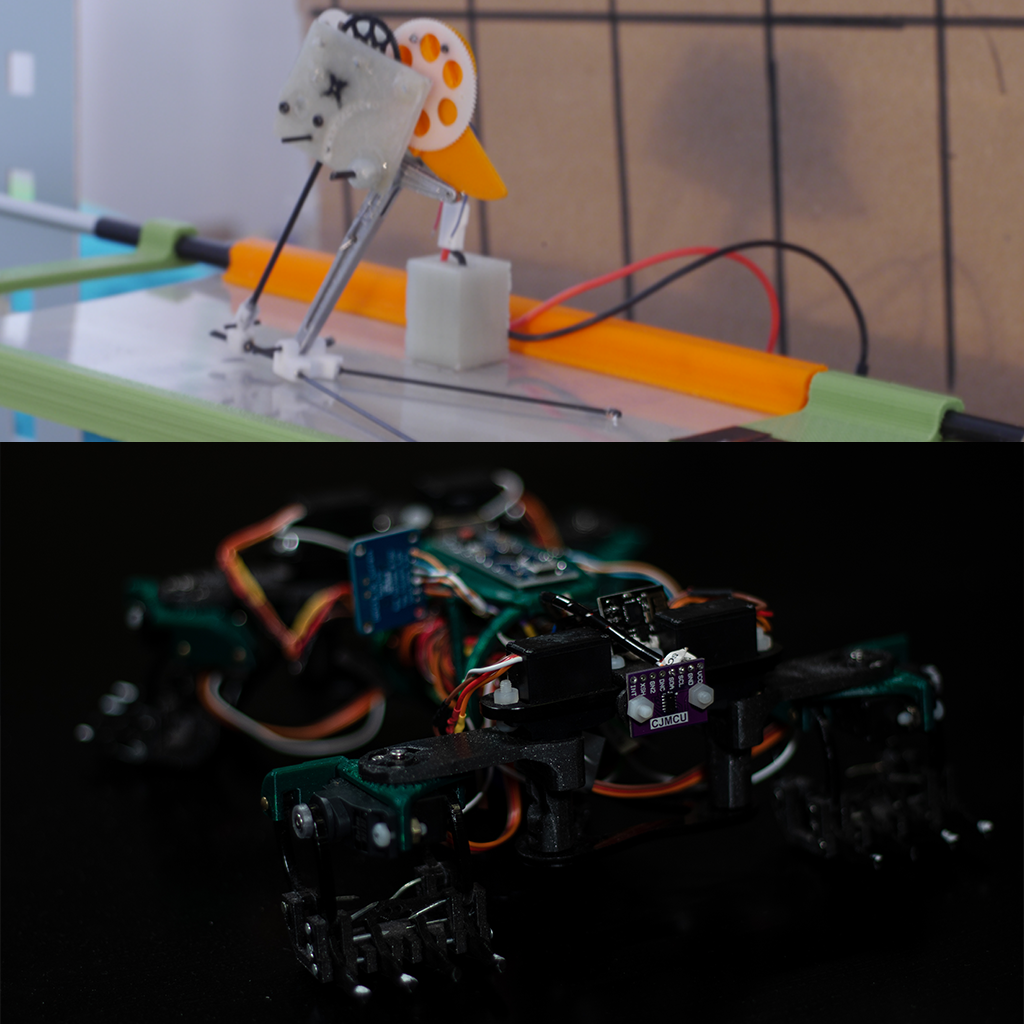
A gap in our knowledge:
Studies which attempt to explore the interaction between selection and constraint are often limited by the availability of species. This is because available species must necessarily form a biased sample. Each species alive today has been successful in the past, and therefore we must assume (at least to some degree) that they are well suited to the habitat which they occupy. Unsuccessful species are likely to have gone extinct. Yet to understand how variation in biomechanical traits can improve the performance of a species, analysis of the less successful strategies is equally important. One way to overcome this limitation is to build biologically mimicking robots and then modify them beyond the limits of what we observe in biological systems. This may reveal why certain combinations of traits are successful and others are not, and explore which biological constraints underpin the limitations to biological optimisation.
A novel research approach:
My lab has developed several biologically mimicking robots through which we can understand character trait evolution. The XGen4 climbing robot is the 4th generation of agile climbing robot capable of climbing rough or compliant, vertical and inclined surfaces. It is based on the running trot of climbing geckos, where the stride is driven via lateral flexion, with diagonal pairs of limbs contacting the surface in unison. Each foot uses a 4-bar linkage system to simultaneously raise and push, or lower and pull the foot, to engage the claws, mimicking the directional dependent adhesive systems of insects and lizards. It is controlled by an onboard Arduino micro system which interacts via an ESP8266 wi-fi board to allow commands to be sent wirelessly to the robot, permitting rapid changes in speed, gait parameters, limb and spine excursion angles. Data is collected via 13 onboard sensors, wirelessly streaming information including distance (speed), current draw (efficiency), motor success rates (accuracy), and orientation (stability), which is then remotely stored. Using this system, we will answer fundamental questions regarding optimal configurations for climbing lizards. For example, we were able to independently modify fore and hind limb angles to show which combinations result in the greatest climbing speed – showing two distinct local optima (Fig 1). Comparing this data to our empirical lizard data reveals a stunning congruence, and the ability to explore how species have moved through the adaptive landscape over evolutionary time.
Computational biomechanics
Combining muscle physiology and biomechanics in virtual computational models to map complex changes in the adaptive landscape.

A gap in our knowledge:
One fundamental goal in evolutionary biology is to understand how size can constrain the evolution of morphological traits and limit performance, which in turn will limit the ability to survive in an ecological niche. Size influences all biological functions from cellular respiration up to how fast an animals can run or climb. Yet to completely understand the influence of size on performance, we require information on the integration of multiple systems, including the nervous, muscular and skeletal systems. When dealing with detailed integrated systems, building physical models such as robots can become difficult and therefore, as I have done in previous publications we rely more on computational and digital models.
A novel research approach:
I (Chris Clemente) have developed a research pathway for the development of these models together with our UK collaborators at the Royal Veterinary College, London (Hutchinson), EU collaborators in Belgium (De Groote) and USA collaborators at Harvard University, Massachusetts (Bishop), using primarily open source software. To do this, CT scans of bones and soft tissue are segmented and imported into the free OpenSim software, via open source CAD software. Muscle 3D paths are constrained using wrapping surfaces and via points to ensure that muscles follow realistic curved or straight paths throughout their range of motion, based on dissection data. Combined with muscle architecture and physiological parameters, I can generate a functional 3D musculoskeletal geometry. With the input of 3D motion capture of movement, and ground reaction forces, the OpenSim workflow allows rapid calculation of inverse kinematics, inverse dynamics and static optimisation. These features allow me to determine joint moments, muscle activations and muscle stresses throughout a stride. The ability to rapidly scale this model both in size and shape allows me to, like our robotic models, understand the limits to performance, via modifying phenotypes independently, without the confounding effects of phylogenetic history.
Biomechanics in the field
Using accelerometers and machine learning to study animal behaviour without watching. What do animals do 24/7?
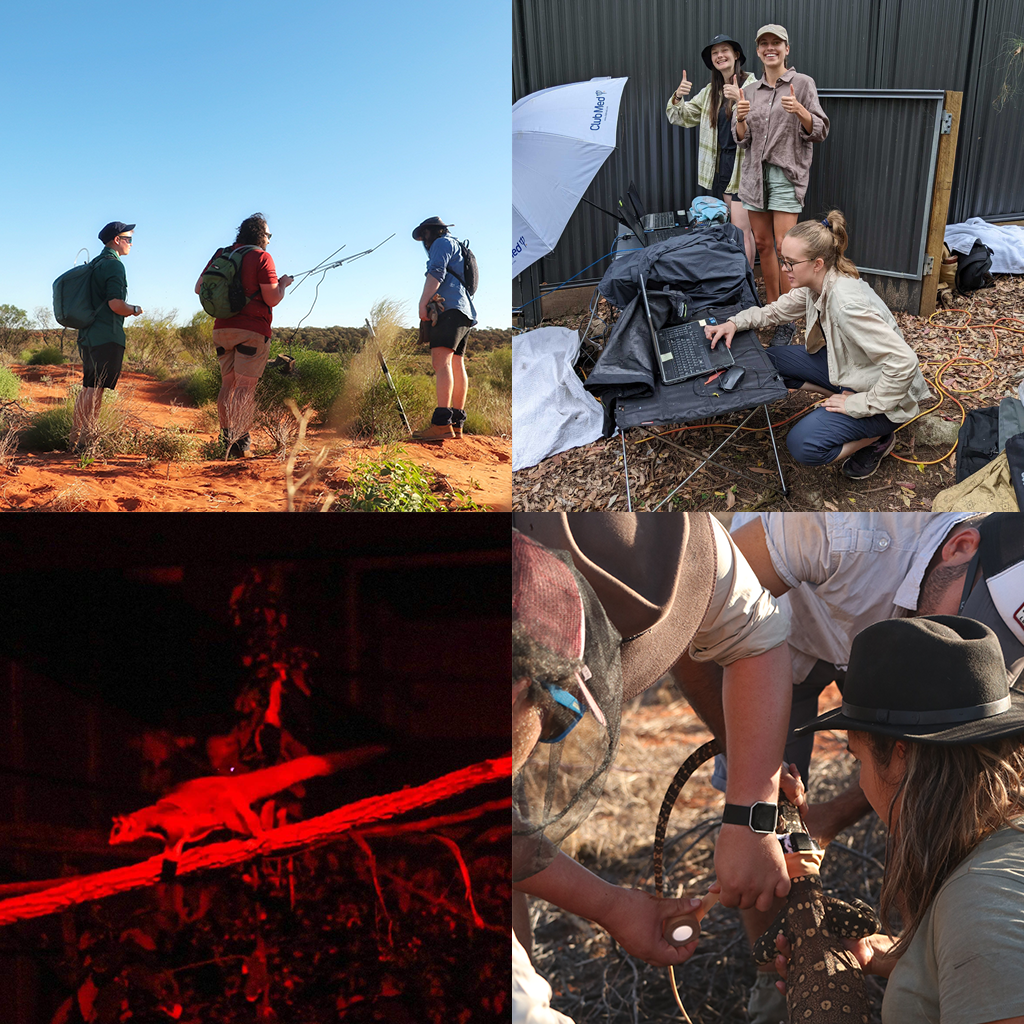
Animals behave differently in the lab vs in their natural habitat. But their behaviour may also be influenced by the fact that we look at them in their natural habitat. Hence, we have initialised a few projects using accelerometers and GPS in combination with camera traps, and machine learning algorithms to find out what these animals do when they’re not watched (but Big Brother was still watching :)). By matching known locomotor behaviour – i.e. feeding, resting, walking, hunting etc. with the responding accelerometer tracks, we can train an algortihm to detect behaviours for the unknown time frames. These type of studies require a lot of field work, which we have done to local wildlife centres or the Australia Zoo, Tasmania, the Outback among others.